Chemistry of Emulsions
Emulsions are a specific type of colloid, or a mixture where microscopic particles are dispersed — without being dissolved — throughout another substance. An emulsion is a specific type of colloid because all the components of an emulsion are liquids.
In creating lotion emulsions, we are combining oil-based liquids and water-based liquids such that the end product appears as a uniform substance. This typically requires a rigorous mechanical force to shear and disperse one liquid into the other. Another example of this type of mixture lies in the kitchen when making a vinaigrette. To effectively combine water-based vinegar with oil, we might use a whisk or blender. In the case of lotions, we can employ a tool such as a stick blender.
Anyone who has combined oil and water knows that, regardless of how much you mix and shake, the vinaigrette will quickly separate into the oil phase (top) and water phase (bottom). This is because vinegar and oil are chemically incompatible. This is true for the water and oil components that we are working to combine in a lotion. Let’s explore why this is the case.
Lotions are Emulsions
In terms of marketing and advertising, lotions are typically lumped into the “cosmetics” category. To a chemist, however, lotions represent a mixture where normally immiscible (unmixable) liquids are combined in a way that evenly distributes one of the immiscible liquids into the other, without dissolving it. We encounter these types of mixtures everyday — in cosmetics, food, medicine, and some household products.
We know lotions as creamy products that feel great on our skin. As it turns out, lotions are a complex mixture of different substances that need to be combined — in certain proportions — in order to stably interact. We can bring the science of lotions back to the classroom in so many ways! For one, they are a great way to talk about intermolecular forces. Additionally, the molecules in an emulsion organize at scales similar to the wavelengths of light, which lead to an opaque appearance. Hence, light scattering and other “physics” concepts can be explored through lotions. You can even bring this back to our biology to understand the interplay of lotions and our skin cells.
Solubility, Intermolecular Forces and, Thermodynamics
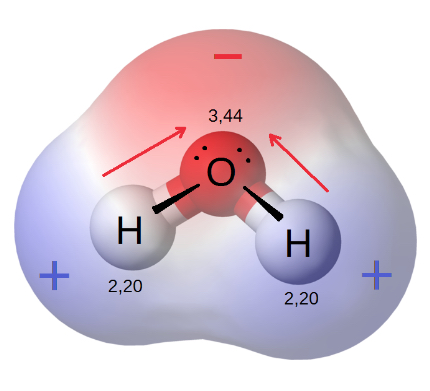
A polar water molecule
The concept of solubility involves examining how a solute interacts with a solvent, which is primarily dictated by the polarity of both the solute and the solvent. As it is understood, polarity is dependent on the electronegativity of each atom in a molecule. Atoms with higher electronegativities (such as oxygen or fluorine) will exert a stronger “pull” on electrons shared in a covalent bond.
For example, let’s look at water. Water is made up of 2 atoms, oxygen and hydrogen, that share electrons through a covalent bond. The electronegativity of oxygen is 3.44, whereas the electronegativity of hydrogen is 2.20. This means that oxygen, with its stronger electronegativity, will exert a stronger pull on the electrons shared between hydrogen and oxygen in the covalent bond, leading to a partial positive charge at the hydrogens, and a partial negative charge at the oxygen.
While covalent bonds do not exhibit a net charge, there can be an unequal sharing of electrons leading to an electric dipole across a bond.
For polar compounds like water, the presence of an electric dipole (partial charge on either end of the molecule) opens up opportunities for dipole-dipole interactions with other molecules. When polar molecules are placed into a polar solvent (such as salt into water), the molecules will orient themselves such that the partial positive charge of one molecule can efficiently interact with the partial negative charge of an adjacent molecule. From a thermodynamics standpoint, these dipole-dipole interactions are energetically favorable, and allow the solute to “dissolve” in the solvent.
When dipole-dipole interactions are extremely strong, such as in the case of water, this is called hydrogen bonding. A great example of this is when we try to dissolve salt (NaCl) in water. Because the sodium and chloride atoms in salt are joined by an ionic bond, NaCl will dissociate into Na+ and Cl– in water, allowing for the dipoles of the water molecule to create hydrogen bonds with the salt atoms.
OK, back to lotions…
In contrast to polar molecules like water and vinegar, the oils we use in our lotions or vinaigrettes belong to a class of molecules called lipids, which are defined by their solubility in nonpolar organic solvents. There are no permanent dipole-dipole interactions in nonpolar compounds, yet lipids exhibit a variety of intramolecular forces nonetheless. Because electrons are in constant motion, the center of a negative charge in a molecule is also in constant motion. On average, the center of the negative charge coincides with the center of a positive charge, resulting in no net charge. However, there are fleeting moments when the center of a negative charge does not exactly coincide with the center of a positive charge, resulting in a transient dipole moment in a molecule. This can then induce a separate transient dipole moment in a neighboring molecule. These attractive forces are called London Dispersion Forces (LDFs).
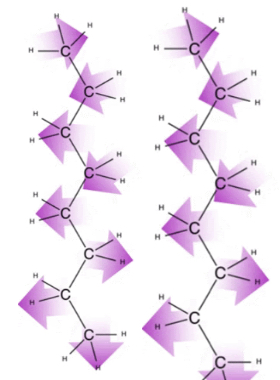
London Dispersion Force Diagram
LDFs are particularly apparent in hydrocarbon chains (found in oils), and get stronger as the number of carbon atoms in the hydrocarbon chain increases. This is reflected by the boiling point of hydrocarbons — the longer the chain, the higher the boiling point. This is because with increased chain length, there is an increase in surface areas that can accommodate increased intermolecular interactions.
Let’s get back to why water and oil do not mix under typical conditions of temperature and pressure. As a polar molecule, water wants to create hydrogen bonds with other polar molecules as this provides a more favorable energy state (the lowest energy state is always the one that is preferred!). Thus, a group of water molecules will orient their dipoles to maximize the amount of hydrogen bonds they can make with each other.
Oils, on the other hand, do not have permanent dipoles (are nonpolar), although they interact with other oil molecules through LDFs. When we introduce oil into the system, water has to make a choice — water molecules can invest the energy to break the hydrogen bonds made with other water molecules to interact with the oil, or they can do nothing (and maintain their hydrogen bond interactions with each other).
Because oil molecules are nonpolar, it would be very difficult for water molecules to interact with oil, so they make the decision to keep bonded with each other. As a result, water molecules remain separate from oil molecules. Due to differences in density, the water will remain at the bottom of the tube (aqueous phase), and oil will “float” on top (organic phase). This is what you see after letting your oil and vinegar salad dressing sit for a while, undisturbed.
So to say that lipids, like oils, are hydrophobic (water-fearing) and that polar molecules are hydrophilic (water-loving) is a bit of a misnomer. It isn’t that oils are afraid of water. Instead, it is more about thermodynamics. From an energy standpoint, it just makes more sense to maintain separateness. However, there are situations when we want oil and water to come together. To make this happen, we have to add something to the system that helps overcome the energy barriers associated with mixing water and oil.