Lipid Background
Lipids are incredible. Defined based on the physical property of (in)solubility, lipids are naturally occurring compounds that are quintessential for life. In our cells, for example, lipids provide physical barriers, act as messengers, provide energy, and even help provide a mechanism for intracellular and extracellular transport. In addition, lipids can be quite tasty!
Lipids Are Defined by Solubility and Intermolecular Forces
As alluded to above, lipids are a class of naturally occurring molecules that are soluble in nonpolar organic solvents, and are not soluble in water. But what are the properties that define lipid solubility? Let’s explore. When talking about solubility, we are basically talking about how a molecule interacts with a solvent—this characteristic is primarily dictated by the polarity of the molecule.
To make this point, let’s start with things that are not lipids. For polar compounds, such as water, the presence of a partial charge on either end of the molecule opens up opportunity for dipole-dipole interactions, or even hydrogen bonding. When polar molecules or ions are placed into a polar solvent, dipole-dipole interactions (or technically ion-dipole interactions for the latter) occur between the solute and the solvent, allowing the compound to dissolve.
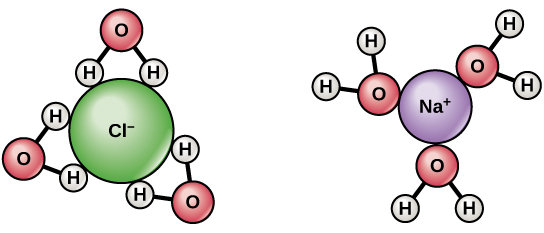
Due to water’s polarity, it can “dissolve” other polar substances, such as table salt (NaCl)
For nonpolar compounds, like lipids, they exhibit dispersion forces with each other, as well as with their solvents. It is unfavorable for polar molecules like water to interact with nonpolar molecules like lipids because the transient charge interactions from the lipid molecules are not sufficient to stabilize the strong separation of charges found on the water molecules.
Dispersion forces are particularly apparent in hydrocarbon chains, and get stronger as the number of carbon atoms in the hydrocarbon chain increases. This is reflected by the boiling point of hydrocarbons — the longer the chain, the higher the boiling point. This is because with increased chain length, there is an increase in surface areas that can accommodate increased intermolecular interactions.
Lipid Classification
Because lipids are largely defined based on solubility in organic solvents, there are a huge number of molecules that are considered to be lipids. Thus it is helpful to sub-categorize lipids. From an organic chemistry perspective, complex lipids are lipid molecules that can be hydrolyzed into smaller, functional fragments (a carboxylic acid and an alcohol). On the other hand, simple lipids are not able to be hydrolyzed into these functional fragments. However, it is important to note that the categories of “simple” and “complex” can be misleading since “simple” lipids can be fairly elaborate in their structure.
Complex Lipids
Complex lipids are considered as such because they contain one or more ester groups — if a strong base (or, in some cases, a strong acid) is added, these ester groups can be hydrolyzed to produce a carboxylic acid and an alcohol. Conversely, the joining of a carboxylic acid to an alcohol through an ester bond is called esterification. For complex lipids, the “carboxylic acid” component is typically a fatty acid (which will be discussed in more detail below).
Examples of complex lipids include triglycerides, waxes, and phospholipids. These are all naturally occurring molecules with important biological functions and are highly regulated in the body. The following schematic is an example of how these molecules can be broken down into their individual parts.
Simple Lipids
As previously mentioned, simple lipids do not readily undergo hydrolytic cleavage due to the lack of a reactive carbonyl or carboxyl group. However, the term “simple” can be a bit of a misnomer. For example, lipid molecules such as steroids do not have a reactive carbonyl or carboxyl group, and thus are not readily hydrolyzed (compared to a triglyceride, for example). However, steroid molecules are fairly intricate, consisting of a tetracyclic ring system with a rigid geometry. In addition to steroids, the other major classes of simple lipids are prostaglandins and terpenes. All three of these simple lipid classes play critical roles in the human body.
Lipids in Our Food
To connect these molecules to foods students are familiar with, the major lipid component in several fatty foods comes in the form of triglycerides — a complex lipid where 3 fatty acid molecules (carboxylic acid) are esterified to a molecule of glycerol (alcohol). Triglycerides are a type of “neutral lipid” since they are relatively inert, storing volatile fatty acid molecules in a way that is more energetically favorable for cells.
While the glycerol portion of the triglyceride remains constant, the fatty acid portion of the triglyceride can vary widely. As mentioned above, fatty acids are carboxylic acids that contain long, unbranched hydrocarbon chains. These chains can vary in length, typically containing between 12 and 20 carbon atoms. Furthermore, fatty acids can be saturated (ie no carbon-carbon bonds) or unsaturated (contains at least 1 carbon-carbon bond). Examples of a saturated and unsaturated fatty acid are shown below.
As you can see from the structural schematics above, the presence of a double bond in the palmitoleic acid dramatically alters the structure of the fatty acid, which, in turn, dramatically alters its melting point. Because of the linear nature of the saturated fatty acid, these molecules can pack more tightly in the solid phase, leading to an increased number of dispersion interactions (and hence the requirement of more energy to break them). Alternatively, the kink resulting from the double bond in unsaturated fatty acids prevents efficient packing, decreasing the overall strength of the dispersion forces holding the fat molecules together.
The general rule of thumb is that saturated fatty acids have a higher melting point than unsaturated fatty acids. Triglycerides containing unsaturated fatty acids are liquid at room temperature whereas triglycerides containing saturated fatty acids are solid at room temperature. This is the difference between an oil and a fat.
Also affecting the melting point is the length of the hydrocarbon chain — longer chains have a higher melting point than shorter chains. This is again related to the strength of the dispersion forces that longer chains afford.
Naturally occurring oils and fats are usually made up of a mixture of triglycerides, meaning that each glycerol backbone can contain up to 3 different fatty acid molecules. However, there are trends. For instance, triglycerides from animal fats typically have a higher percentage of saturated fatty acids compared to triglycerides extracted from plants oils. As such, they also have different impacts on human health.
Trans Fats
When speaking of the double bonds in naturally occurring unsaturated fatty acids, we are usually referring to a cis double bond formation. Cis bonds are formed in nature (as opposed to trans bonds) because of the enzymes responsible for desaturation reactions — reactions that transform a saturated carbon-carbon bond into an unsaturated carbon-carbon bond — operate in a way that only results in cis bond formation.
While unsaturated fatty acids are generally considered to be “heart healthy,” the presence of one or more double bonds in the hydrocarbon chain makes these molecules more susceptible to autooxidation (reaction of oxygen with the double bonds). If enough autooxidation occurs, the oil will go rancid — this is why food oils tend to have a much shorter shelf life than fats (saturated fatty acids are more stable).
To address this issue, and prevent food waste, food scientists implemented a method to partially hydrogenate unsaturated oil mixtures until the desired texture was obtained. By reducing the double bonds in an unsaturated fatty acid mixture, the melting point can also be altered. This change in the fatty acid physical property favors some cooking applications. For instance, Crisco, which was widely used for baking, is actually made up of partially-hydrogenated cottonseed oil. Before hydrogenation, cottonseed oil is liquid at room temperature; after hydrogenation, it is a solid at room temperature, but it melts upon cooking. Margarine is made in a similar fashion. This is a great demonstration of the role of intermolecular forces in determining the physical properties of a substance.
Hydrogenation is actually a very energetically expensive reaction. However, by using a metal catalyst (usually Nickel), the activation energy is significantly lowered, making the reaction scalable. Furthermore, partially-hydrogenated vegetable oils are much cheaper than animal-derived fats, so this process also benefits the pocketbooks of consumers.
While partial-hydrogenation sounds like a good idea on paper, the metal catalyst can also induce isomerization of the cis double bond in a fatty acid, converting it to a trans double bond. It is estimated that the hydrogenation process used in food production produces a mixture containing 10 – 15% trans saturated fatty acids. It was recently discovered that trans fats can impact our cardiovascular systems by raising the amount of LDL (low density lipoprotein, aka “bad cholesterol”), and increase the risk of heart disease. As a result, the amount of trans fats in food must be included on the food label, as per FDA requirements.