Soil Chemistry
Nutrients
There are 17 essential nutrients for a plant’s growth. Three of these are derived from air and water, and account for 94% of a plant’s weight. These are carbon, oxygen, and hydrogen. The other 14 nutrients must come from the soil.
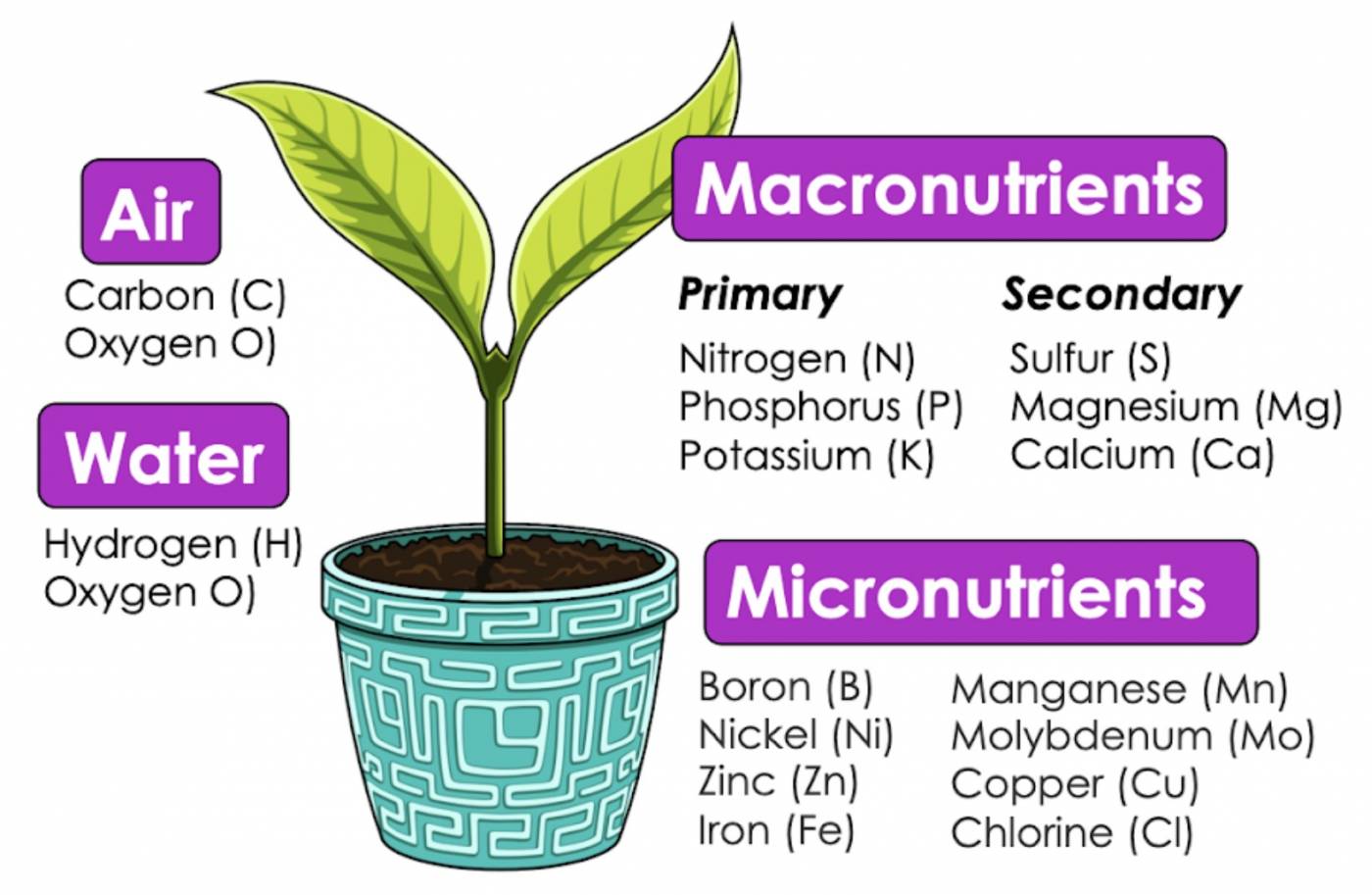
Figure 1: The 17 essential nutrients for plants. Macronutrients are consumed in extensive amounts, with primary macronutrients being the most heavily consumed. Micronutrients are required in trace amounts.
Nutrients in soil are divided into macronutrients (needed in larger quantities) and micronutrients (needed in smaller quantities). The figure below shows the elements that are classified as macro- vs micro- nutrients.
Roots can only absorb nutrients dissolved in water. The nutrients that are not dissolved in water can be found in solid form (newly applied fertilizer granules), in adsorbed form (bound to soil particles like clay and organic matter, Fig. 4), or in chemical compounds (decomposition products released by microorganisms).
If roots can only absorb dissolved nutrients, how does the plant make use of those locked away? Well, the soluble and insoluble forms of nutrients are in equilibrium with each other.
Think of the soil as a bank for plants. The dissolved nutrient pool is like a checking account and the undissolved pool is like a savings account. In the checking account, funds are immediately available. If the checking balance is low, transfers are made from the savings account to the checking account. Oppositely, if the checking account becomes full of money, some can be transferred to savings for storage. In the same way, when there is an excess of nutrients in the soil water, some can bind to soil particles and some can react to form insoluble minerals for storage. Here, they remain temporarily unavailable until they are needed again, at which point they can desorb or redissolve into the soil water.
Many of the nutrients in soil are in a cationic form, or positively charged. Electrostatics has a lot to do with the way these nutrients “stick” to the soil minerals. Later we will discuss why different types of soil have differing abilities to retain nutrients.
Soil pH
pH is key to healthy soil and healthy plants. pH is a measure of the concentration of hydrogen ions in a soil solution, and describes a soil’s acidity. The lower the pH, the higher the acidity of the soil, and the higher the concentration of H+ ions in the soil solution. pH is a logarithmic scale; this means that soil at pH 5 is 100 times more acidic than pH 6, and 1000 times more acidic than pH 7. A small change in pH results in a large change in acidity.
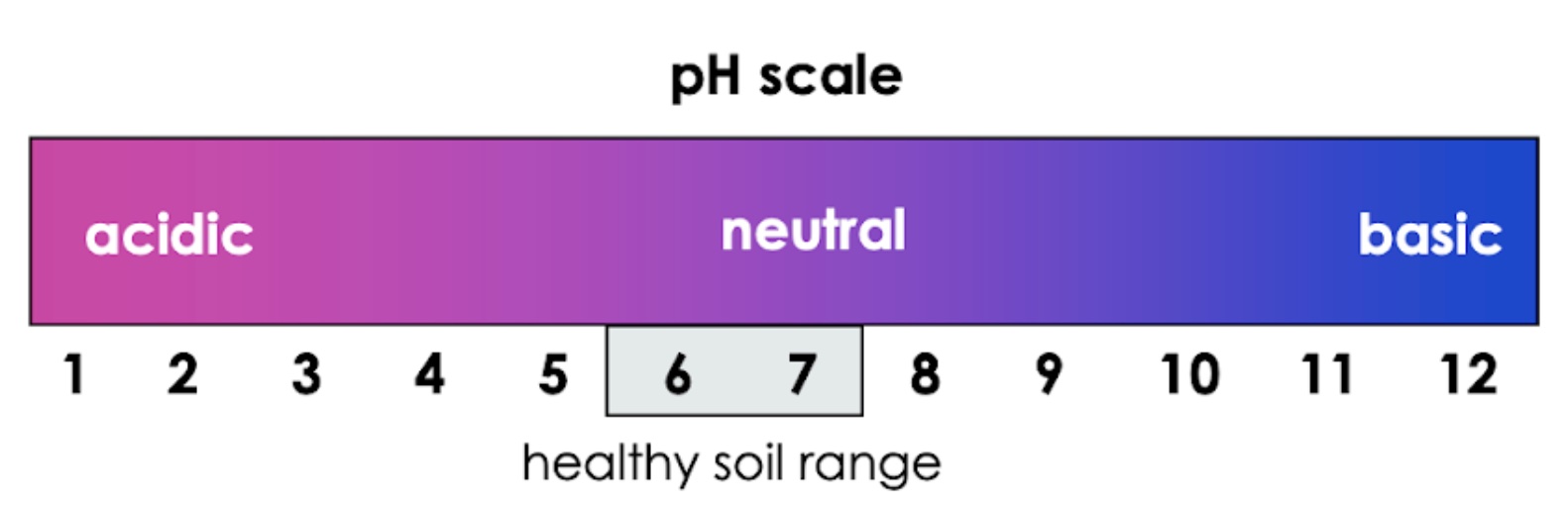
Figure 2: pH scale showing the optimal pH range of most plants
Most plants grow best in soils with a pH close to neutral (pH 5.5 – 7.5). Outside of this range, plants quickly begin to die. Let’s learn why this is.
The reason pH is so important is that the soil’s pH affects the ability of plants to access nutrients. Roots can only take up nutrients in a dissolved form – solid nutrients remain in the soil, inaccessible to plants. The availability of some plant nutrients is greatly affected by pH – a nutrient may be soluble at some pHs (making it available to plants) but insoluble at others.
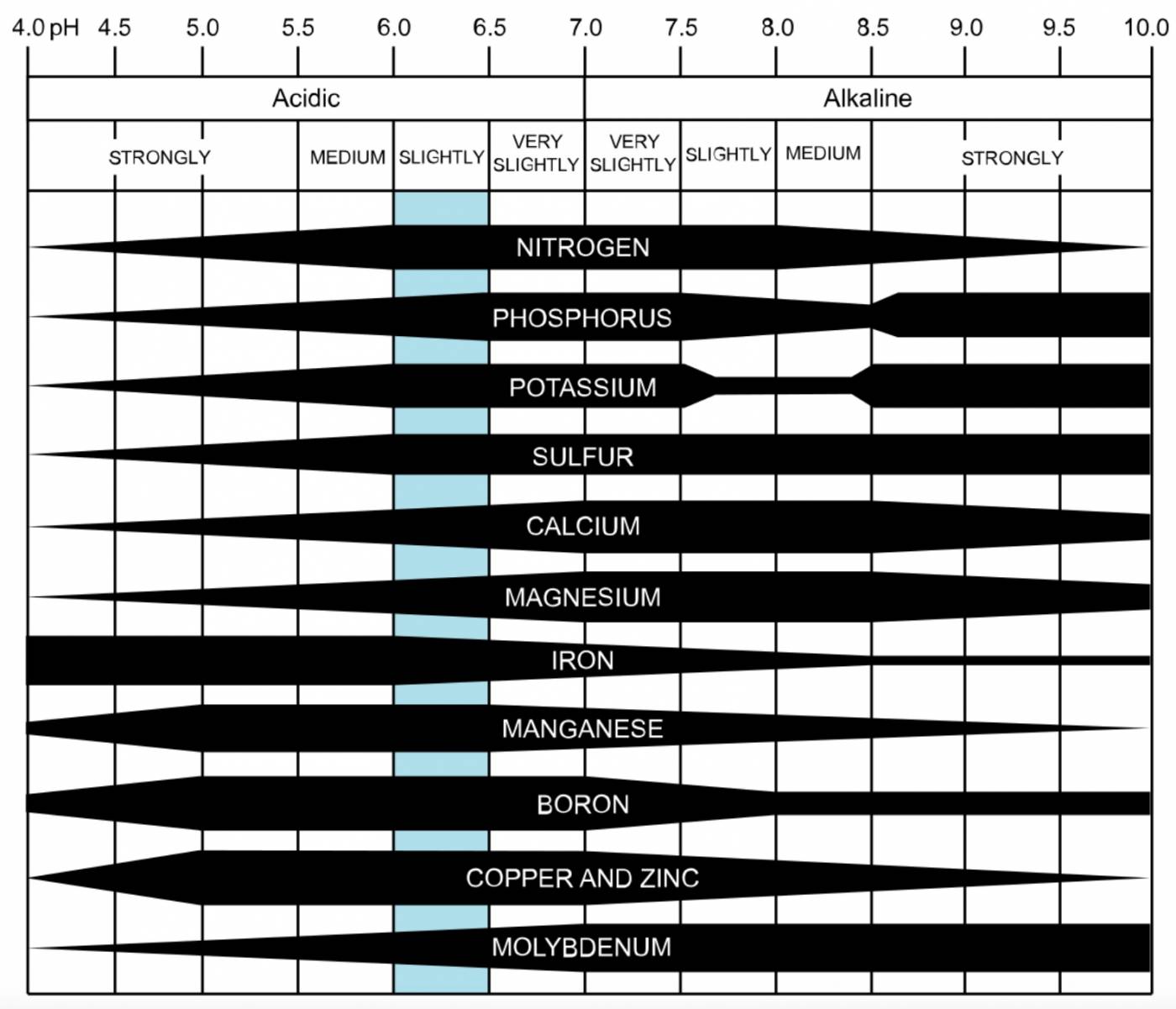
Figure 3: Soil nutrient solubilities are dependent on pH. The thickness of the black bar represents solubility – the thicker the bar, the more soluble (and the more plant-available) the nutrient. The blue shaded column shows the “sweet spot” that has the optimal soluble concentrations of each nutrient.
Phosphorus is an example of a nutrient that is highly dependent on soil pH. At alkaline pHs (>7.5) phosphate ions react quickly with calcium and magnesium to form less soluble compounds. At acidic pHs, aluminum and iron are made soluble, and react with phosphate ions to again form less soluble compounds. Phosphorus becomes most soluble between pH 6 and pH 7 because reactions forming insoluble phosphate products are limited in this range. Thus, tight control over the pH of soil helps roots access essential nutrients while preventing roots from taking up nutrients at harmful or toxic concentrations.
The chart in Figure 3 shows several nutrients and their relative solubilities along the pH scale.
Farmers and gardeners can measure the pH of their soil by making a 1:1 soil-water slurry and using a pH probe to monitor the pH of the slurry. If a soil is too acidic, a common fix is to add lime (calcium carbonate) which reacts with excess H+ ions to lower the pH.
Soil as a buffer
When measuring the pH of soil, you’re really measuring the concentration of hydrogen ions that dissolve right away in water. However, not all hydrogen ions are immediately soluble. Like nutrients, some H+ ions are adsorbed to solid matter in soil (in other words, “stuck to the dirt”), and therefore do not contribute to the soil’s active acidity. These “reserve” hydrogen ions make up the soil’s exchangeable acidity. These H+ ions are termed exchangeable because they are able to switch places with other cations in solution (e.g. nutrients). The H+ ions leave their spot on the solid matter and become soluble themselves, while the cations get adsorbed, or vice versa (Fig. 4).
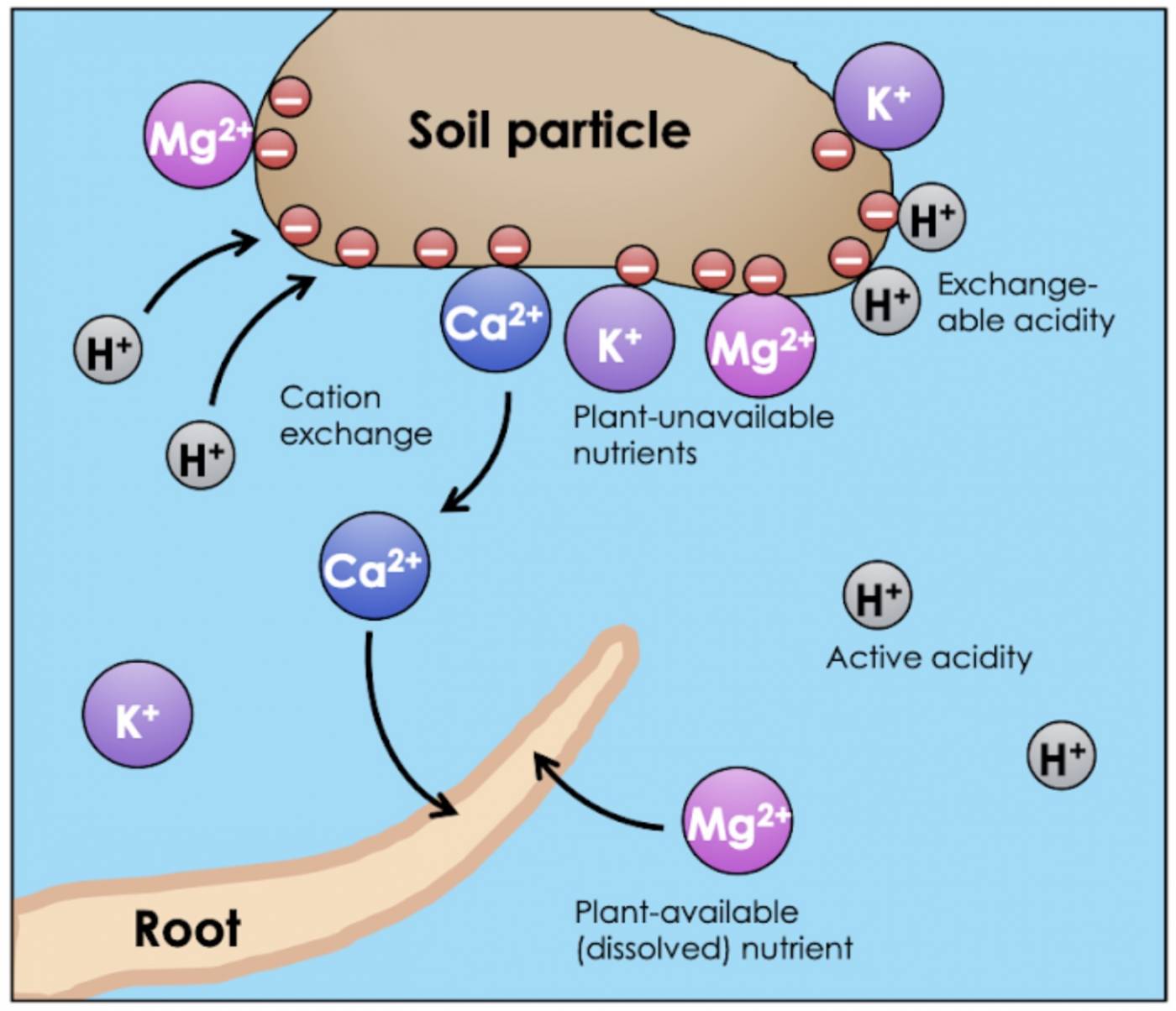
Fig 4: Cation exchange between soil water and a soil particle (clay or organic matter). Cation exchange is the exchange of cations (here, H+ and Ca2+) between soluble and insoluble forms. Roots can only absorb dissolved nutrients. Dissolved H+ ions contribute to the active acidity of the soil water; adsorbed H+ represents exchangeable acidity.
The exchangeable acidity (adsorbed H+) of soil increases the soil’s buffering capacity, or the ability of the soil to resist a change in pH. If a soil becomes too basic, the “reserve” exchangeable H+ ions will become soluble, replenishing the acidity. Likewise, if the soil becomes too acidic, dissolved H+ ions can exchange with adsorbed cations, decreasing the active acidity of the soil water. As we’ve seen, fluctuations in soil pH can cause dramatic nutrient imbalances, so the ability to buffer pH changes effectively is an important property of soils.
The amount of positive charge the soil’s solid matter can hold is called the cation exchange capacity (CEC) of the soil. A high CEC means a soil has a high buffering capacity (lots of exchangeable H+ can be adsorbed). A high CEC also means more nutrients can be stored and ultimately released for plant uptake. Potassium deficiencies are common in soils with a low CEC because they can’t hold on to their cationic nutrients as well, resulting in K+ leaching.
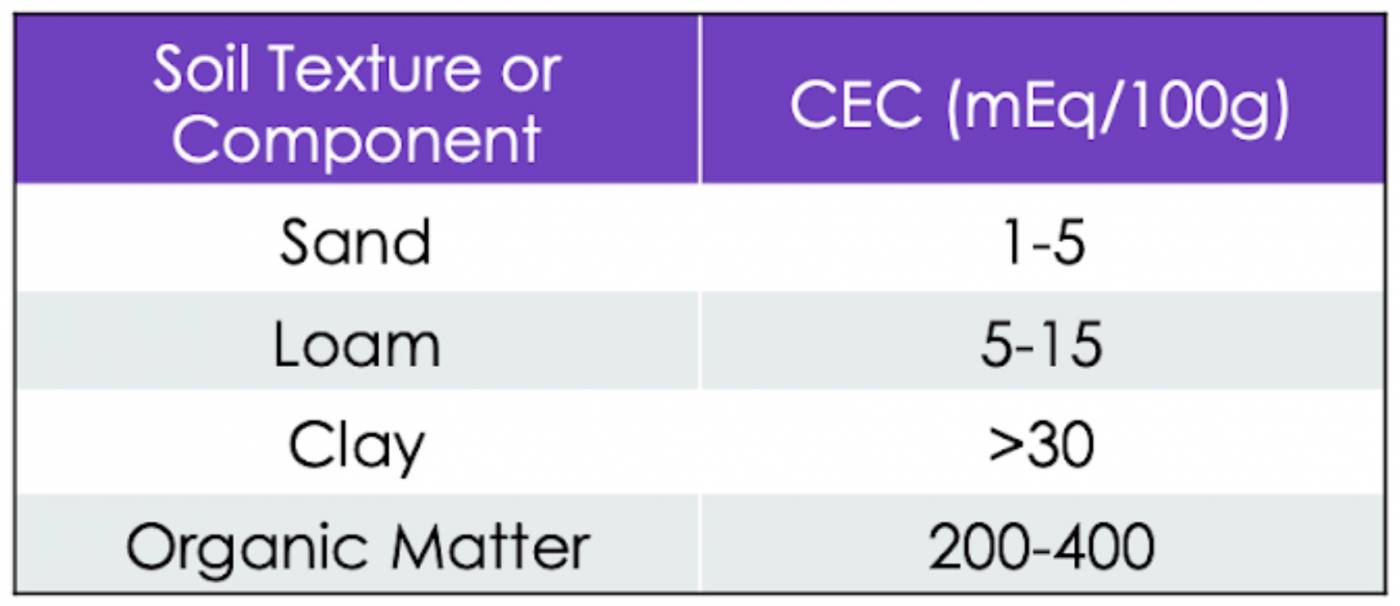
Table 1: Cation exchange capacity for different types of soil, reported in milliequivalents of charge per 100 grams of soil.
If you’ve gotten to Know Soil Physics, you may have a guess at which soil mineral greatly enhances CEC, buffering capacity, and exchangeable acidity. If you guessed clay, you got it! Clay has the highest surface area-to-volume ratio of all the mineral matter in soil, and its surface is covered in negative charges, providing lots of adsorption sites for cations. Thus, soils with a higher clay content will be able to hold more nutrients and control pH levels more effectively. However, there is another soil solid that has an even higher (far higher) ability to hold cations than clay – organic matter.
Organic Matter
Soil organic matter (SOM) is the plant, animal, and microbial residues in soil at various stages of decomposition. The word “organic” indicates that these chemical species are carbon-based and are derived from living things. For this reason, the term soil organic carbon (SOC) is used in some contexts. In fact, organic matter has approximately a 100:10:1 ratio of the elements C:N:P, making carbon the dominant element in SOM. Organic matter makes up a relatively small proportion of soil (~5%), but has disproportionately large impacts on soil health.
Organic matter has recently been emphasized as a continuum of decomposition products, from intact tissues of plants and animals to a highly decomposed mixture of chemical species called humus. However, to aid our thinking, we can categorize organic matter into two pools:
- Active organic matter
- Stable organic matter
Active organic matter is the fraction of OM being rapidly decomposed by microbes. These are simple chemical species (sugar, starches, proteins) that come from cellular debris and are easily digestible; consider this OM “fresh meat” for microbes. During the decomposition process, nutrients such as nitrogen, phosphorus, and potassium are released in a plant-available form. Because of this, the amount of active organic matter in soil serves as a good indicator of soil fertility.
Stable organic matter, also known as humus, is so well-decomposed that it is impossible to tell what it was to begin with. This pool of OM is thought to be composed of highly complex chemical compounds that form by integrating breakdown products from plants and those from microorganisms. The complex structure of humic substances make them difficult for microorganisms to digest, resulting in long residence times in soil. While humus does not provide food for microbes, it serves other physical, chemical, and biological functions, and is considered one of the most important components of healthy soil.
Humus has an exceptional ability to retain nutrients and water because it has negatively charged chemical groups which electrostatically “stick” to cationic nutrients and polar water molecules. The process of humification (successive decomposition towards humic substances) has the following effects: 1) colors the soil darker (Fig. 5), 2) increases water holding capacity, 3) improves soil structure by acting as “glue” that clumps mineral matter into aggregates, which 4) increases porosity and 5) creates habitats for soil biota. It also 6) sequesters carbon below ground and out of our atmosphere and 7) buffers against pH changes and 8) provides food for microorganisms and nutrients for plants.
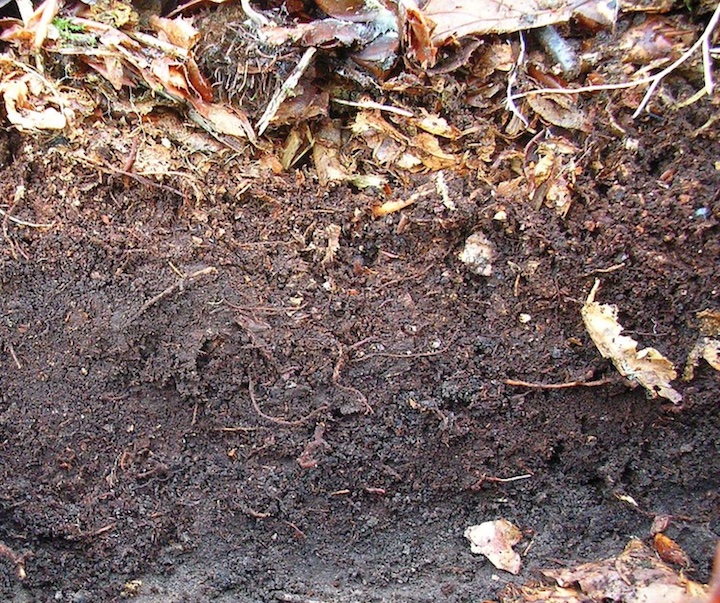
Fig. 5: Humus-rich soil. The dark color indicates the presence of humus.
While all of these humification effects are well-accepted, humus is still one of the most enigmatic areas of soil science. In recent years, there has been a paradigm shift regarding the chemical properties and composition of humus. Leaders in the soil science field are now suggesting that humus is not actually as long-lasting and stable in soil as was previously thought, and rather, its persistence in soil is more due to its physical separation from decomposers rather than its chemical recalcitrance. This new way of thinking may lead to new models of soil organic matter that better explain our observations, and ultimately will impact the way we manage and promote the health of our soils.
For more explanation of this paradigm shift, see https://www.mofga.org/resources/soil/humus-is-dead-long-live-humus/ or this Nature article for a deeper dive, https://www.nature.com/articles/nature16069