What makes you move?
Have you ever wondered how your muscles know how to move when you are walking, dancing or smiling? The human body has three systems acting together to make it move: the muscular, skeletal and nervous system. The muscular system consists of over 600 skeletal muscles that allow us to move around! Some muscles allow you to bike or dance, while others aid in chewing, or blinking. Muscles are like an elastic band; they can stretch and shape depending on the performed movement.
For example, when you bend your arm at the elbow, the muscle called the “bicep” contracts, and its shape gets shorter and thicker. When it stretches, it relaxes and its shape gets longer and thinner.
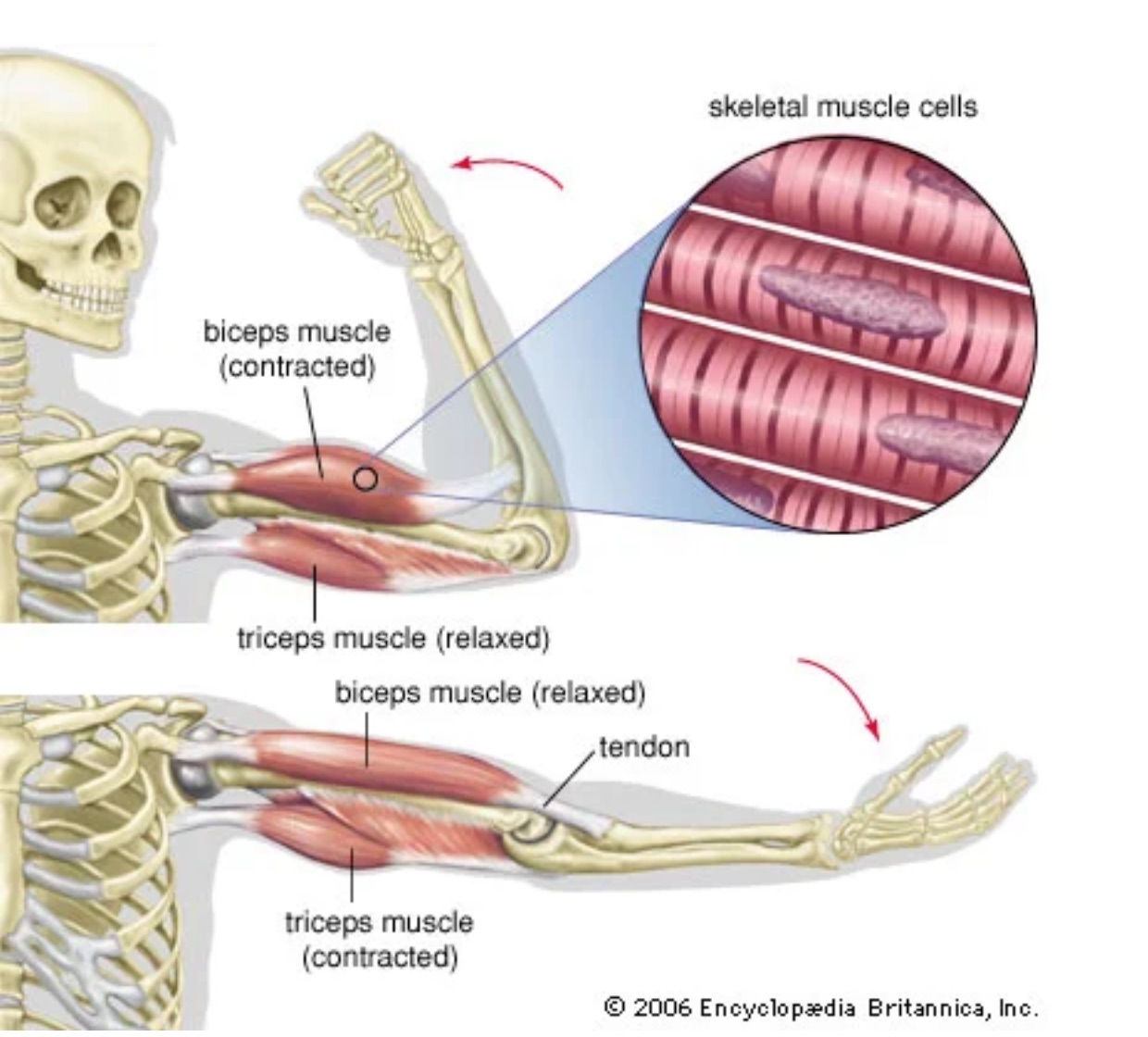
Contraction and relaxation of the biceps and triceps muscles. Taken from https://www.britannica.com/science/biceps-muscle.
Skeletal muscles are attached to our bones, through tendons, which are strong cords of connective tissue. When a muscle contracts or relaxes, it moves the bone into a different position. But how do muscles know when and how they are supposed to move? These movements are actually controlled by our nervous system, and more specifically our brains (1)!
The nervous system meets the muscle at the neuromuscular junction. When neurons at the neuromuscular junction fire, acetylcholine, an excitatory neurotransmitter, is released. The muscle fibers respond to acetylcholine by contracting and consequently causing the muscle to move (2). Through this pathway, neurons within certain brain areas control muscle movement.
It took several years for scientists to discover the areas in the brain that control movement. In around 1800, John Hughlings Jackson became interested in the brain cortex as a center for movement initiation. Jackson was studying epileptic seizures, and hypothesized that abnormal electrical activity in a partial seizure might move through the cortex and cause corresponding abnormal body movement. In 1870, Gustav Fritsch and Eduard Hitzig, using electric micro-stimulation, provided support for Jackson’s hypothesized “cortical motor region”. By electrically stimulating the cortex of dogs they produced twitches of small groups of muscles on the opposite side of their bodies. The location of the electrical stimulation determined which body part would move. Located lesions in the cortex will impair movement in particular body parts. Later, David Ferrier, replicated the findings, and tested the stimulation in other animals. By using alternating current and longer stimulating intervals, he made the animals display full, complex movements, similar to how they would move in real life (2).
Until the end of the XIX century, it was evident that the “movement function” in the cortex was localized in one side of the brain, and that neurons -within this location, project to neurons in the spinal cord on the other side. At the same time, the neurons in the spinal cord project to the muscle fibers where they release acetylcholine. This pathway is called the “direct motor pathway” by which neurons in the motor cortex control muscles. However, there are also indirect pathways where motor neurons send their outputs through intermediate brain areas to the spinal cord (more on that, here and here.)
A fundamental question is how the neural activity of cortical motor neurons encodes instructions for controlling our body movements. It was not until 1950 that scientists could assess this question by recording the neural activity in awake and moving animals. Studies revealed single-neuron responses in the motor cortex are complex. Hence, there is little consensus regarding what features or aspects of the movement the neurons in the motor cortex are encoding. For example, is the number of action potentials of a neuron or a population of neurons encoding the parameters of the movement to be executed, e.g. the velocity, direction, or position; or is it encoding the forces and torques generated across the single joints to move the muscle. Some studies favor the representation of higher-level movement parameters (e.g. arm velocity), while others suggest that the neural activity relates more to the driving of the muscle activity. This controversy has yet to be solved, and recently, scientists have pivoted to a different question:
What principles might guide the generation of the observed patterns in the motor cortex?
Fundamental contributions from a dynamical system framework and population activity perspective have helped in understanding the neural dynamics in the motor cortex. Under this framework, a neural population constitutes a dynamical system. A dynamical system is a system in which a mathematical function describes the time dependence of a point in an ambient space. For example, the mathematical model describing the swinging of a pendulum, or the flow of water in a pipe are examples of dynamical systems. When people talk about a dynamical system framework to understand cortical motor responses, it means that the neural population in the motor cortex constitutes a dynamical system that through its temporal evolution, performs computations to generate and control movement. For this to happen dynamical features should be present in the neural population response. Studies from the Shenoy lab have shown that the motor cortex uses the rhythm across the population of neurons to generate patterns that provide different types of information (3). Imagine each neuron is behaving like a player in a Big band, and when the rhythms of all the players are summed over the whole band, a signal for a movement based on all the neurons firing at that given moment is generated (3,4). Although this framework has been extremely successful in predicting several effects of the population dynamics on motor behavior, many questions remain unanswered, and scientists are still working on unraveling the mysteries of the motor cortex (2,3)
Fortunately on the pragmatic side, technological developments have allowed us to extract motor commands from brain signals to control external devices and improve motor recovery in patients, through brain computer interfaces (BCI) (5,6). Nowadays, BCI can turn speech -related activity into text, enabling paralyzed patients to communicate at a rate of 62 words per minute,7, approaching the speed of natural conversation (160 words per minute)!
Let’s practice!
- Smile, which muscle is the most responsible for your smile? Which nerve controls it? Can you control this muscle?
- Breath, what kind of muscles are you using for this? Can you control them?
- Close your eyes and listen to your heart, what kind of muscles is your heart using to move? Can you control them?
- Based on the above, how many types of muscles humans have?
Food for Thought
- Do all animals use muscles to move?
- What are muscles made of?
- How does a snake move if it doesn’t have legs?
- Intracortical brain machine interfaces (BMI) can use the neural activity of which brain area to move robotic arms?
- https://www.youtube.com/watch?v=eRSEAP4M69s&t=1s
Sources
- How Do Our Bodies Move? SciShow Kids. https://www.youtube.com/watch?v=j918PoWWaB0
- Grace, Lindsay (2021) Models of the Mind: How Physics, Engineering and Mathematics Have Shaped Our Understanding of the Brain, Bloomsbury Sigma, Chapter 8: Movement in Low Dimensions. Pag. 215-246.
- Vyas, S., Golub, M.D., Sussillo D., & Shenoy K.V. (2020), Computation Through Neural Population Dynamics, Annual Review of Neuroscience, 43: 249-275.
- Carr, L. The Neural Rhythms That Move Your Body (2012). The Atlantic. https://www.theatlantic.com/health/archive/2012/06/the-neural-rhythms-that-move-your-body/258094/
- Gupta A, Vardalakis N. & Wagner FB. (2023) Neuroprosthetics: from sensorimotor to cognitive disorders, Communications Biology, 6:6(1):14.
- https://www.youtube.com/watch?v=eRSEAP4M69s&t=1s
- Willett F.R., Kuz E., Fan C., Avansino D., Wilson G., Choi E.Y., Kamdar F., Hochberg L.R., Druckmann S., Shenoy K & Henderson J. (2023) A High-performance speech neuroprosthesis, Biorxiv, https://doi.org/10.1101/2023.01.21.524489.